On the desolate Antarctic peninsula, a colony of penguins creates a hub of biodiversity. One may ask, how exactly do those aquatic birds help maintain and enrich the variety of different kinds of organisms from plants and animals, to a wide range of insects and micro-organisms that live on our planet? The answer is quite intriguing. Scientists from the University of Science and Technology of China ...[Read More]
Predators or gardeners: how penguins fertilise Antarctica’s biodiversity
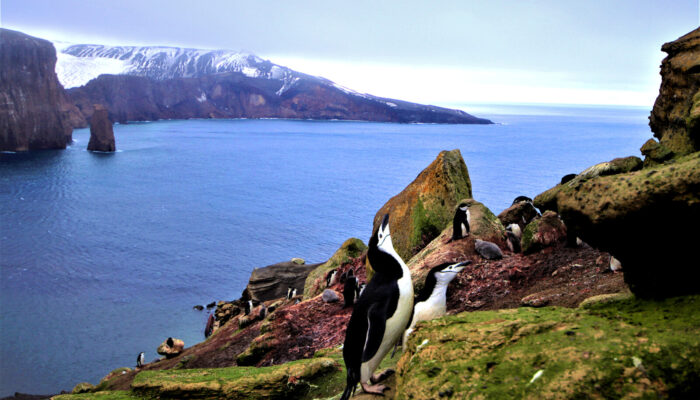